Solar Weather
If you’re looking to catch the solar eclipse’s impact on the ionosphere using your ham gear, here’s a streamlined way to do it with WWV signals:
Gear Up:
You’ll need:
- Your trusty HF rig, tuned to WWV’s familiar frequencies (10, 15, and 20 MHz).
- A way to gauge signal strength – your rig’s S-meter will do just fine, or you could go digital with an SDR setup.
- A log (paper or digital) to track what you hear and see in terms of signal behavior.
Execution Plan:
- Pre-Eclipse Recon: Dial in WWV frequencies well before the eclipse to get a baseline. Knowing what’s normal helps you spot what’s not.
- Eclipse Watch: As the moon starts to bite into the sun, keep an ear on the changes. Log the signal strength and quality across WWV frequencies as you go through the eclipse phases.
- The main show is at totality, but the lead-up and wind-down are worth your attention too.
- Post-Eclipse Debrief: Stick around after the celestial event. How quickly do signals return to their pre-eclipse state? This can tell you a lot about ionospheric recovery.
Anticipated Radio Phenomena:
- Clearer Signals: As the D-layer’s absorption drops, some signals might punch through clearer than you’re used to.
- Unpredictable Fading: Ionospheric instability could make signals flutter in ways that defy normal propagation logic.
- Band Behavior: Watch how different bands react. Lower bands might suddenly outperform higher ones, or vice versa, depending on the time of day and your QTH.
In short, use the eclipse as a unique opportunity to study propagation in real-time. It’s a rare event that can offer valuable insights into how celestial phenomena influence our hobby. Plus, it’s a great excuse to spend more time on the air!
The phenomenon of a solar eclipse has pronounced impacts on radio wave propagation, especially at frequencies like 18 MHz. Here’s a detailed exploration of these effects:
Ionospheric Dynamics: The ionosphere plays a crucial role in the reflection and absorption of radio waves, facilitating long-distance communication. This atmospheric layer is significantly influenced by solar radiation. During a solar eclipse, the reduction in solar radiation alters the ionosphere’s behavior, especially noticeable in the D-layer and E-layer:
- The D-layer, which typically absorbs high-frequency (HF) radio waves, witnesses a decrease in its absorptive capacity due to diminished solar radiation. This reduction allows HF waves to travel further with less attenuation.
- The E-layer, critical for HF wave reflection, undergoes alterations in electron density. These changes can modify how and where radio waves are reflected back to Earth, affecting signal paths.
Propagation Enhancements: At 18 MHz, the eclipse-induced ionospheric modifications can enhance propagation characteristics. With lower absorption in the D-layer and altered reflection properties in the E-layer, radio signals at this frequency enjoy increased range and reliability, potentially improving long-distance HF communication channels.
Anomalies and Variabilities: However, these conditions can also introduce anomalies such as irregular signal fading, heightened background noise, and occasional loss of signal. These effects are particularly noticeable along the eclipse’s path edges, where the ionosphere transitions sharply between shadowed and sunlit states.
Temporal Factors: The impact on 18 MHz propagation is most pronounced during the totality phase of the eclipse when the Sun is completely obscured by the Moon. The extent and nature of propagation changes are also influenced by the eclipse’s duration and the specific time of day it occurs, affecting the ionosphere’s responsiveness to such an event.
It’s crucial to understand that these effects are not uniform and can vary based on geographic location, time of day, and atmospheric conditions. Monitoring and analyzing ionospheric responses during a solar eclipse can offer invaluable insights into radio communication strategies and propagation phenomena.
Latest Solar Image
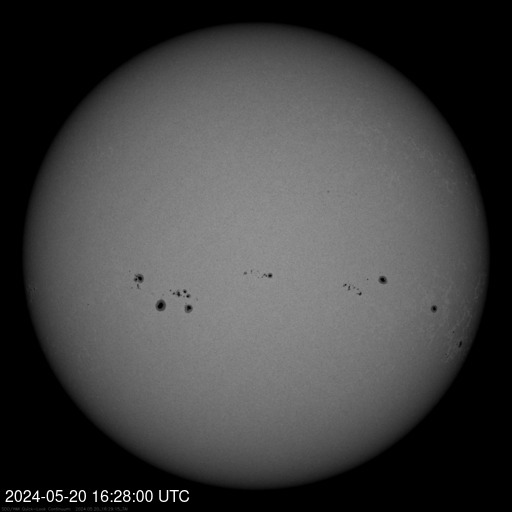